Low Earth Orbit (LEO): An Overview
What is a Low Earth Orbit? What do we use it for? Both the military and civil sectors exploit this orbit for observation, reconnaissance and communication purposes.
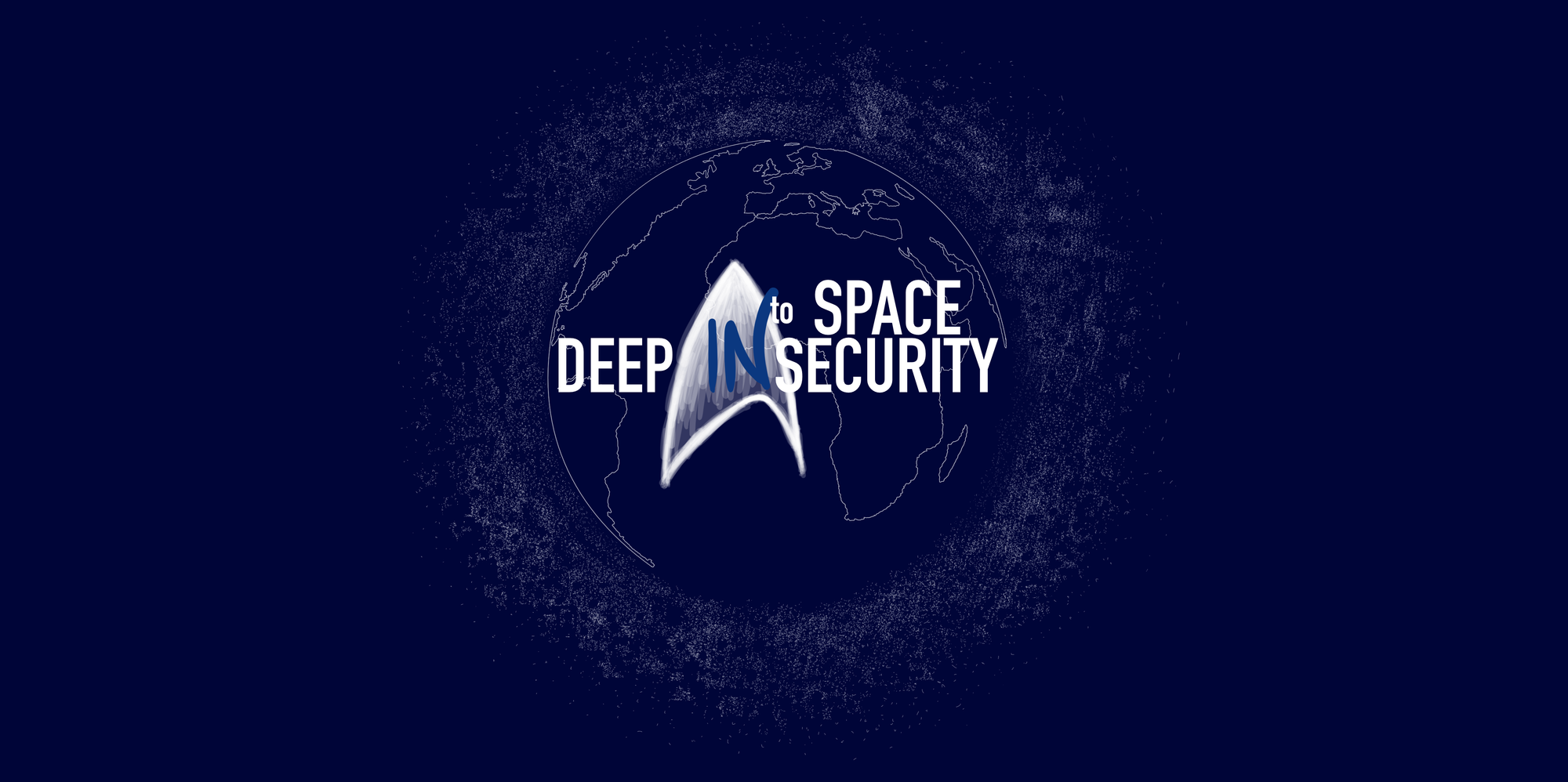
1. What is a Low Earth Orbit (LEO)
Low Earth orbits (LEO) are orbital paths around the Earth defined by altitudes ranging from approximately 100 to 2,000 kilometres (Figure 1) (Defense Intelligence Agency, 2019, p.12).
Orbits up to an altitude of around 1,000 km are, however, the most widely used, as altitudes beyond this threshold are subjected to significant levels of radiation and charged particles - space weather (Wright et al., 2005, p.40).
In recent years, advancements in space launch technology have opened up opportunities to utilise what is now termed as Very Low Earth Orbit (VLEO), typically denoted by altitudes below 450 kilometres (Crisp et al., 2021, p.476).
These orbits were formerly impractical due to aerodynamic drag, resulting in the decay of satellites within a few years. As a result, frequent replacements were required, thereby significantly increasing maintenance costs.
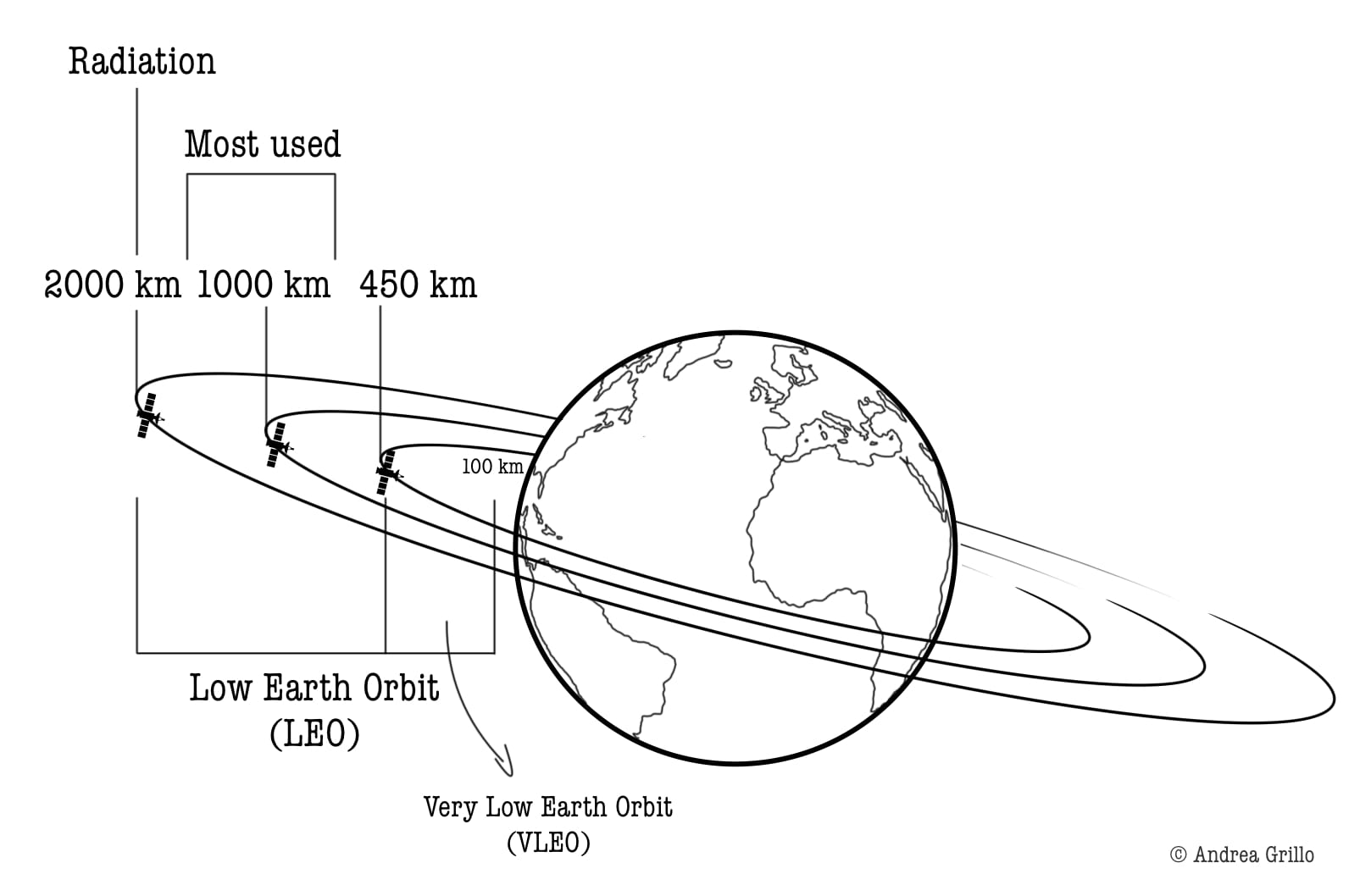
2. LEO Applications
Low Earth Orbits are mainly used for observation and reconnaissance satellites (ISR Satellites) which need high-resolution images –
“military observation to include photographic, imaging, and radar satellites, and resource management satellites that can take a variety of multi-spectral images” (Dolman, 2005, p.56) —being closer to the subject
– and manned flight missions.
These missions are particularly suitable for such an orbit where they do not need continuous worldwide coverage (not time-critical missions).
Since the orbital period is only 90 minutes (14-16 complete orbits per day), a time-critical mission would require a large constellation of satellites (Figure 2) (Wright et al., 2005, p.41).
Requiring a large constellation of satellites for continuous coverage, one might think that such orbits are not suitable for telecommunications satellites. While this impediment does exist, it is important to consider the accompanying benefits.
- Firstly, when defining latency as the time it takes to transfer round-trip (round trip time - RTT) computer data from Earth (source) to a satellite (destination) and back to Earth, this value in such orbits stands at only 0.005 seconds (RTT=5 ms). Latency is indeed influenced by factors such as network congestion, processing time, and distance travelled. Given their low altitude, round-trip transmission is thus extremely short, also eliminating the need for echo control and other treatments. Lower latency indicates faster response times and improved performance in communication systems.
- Secondly, through a high orbital inclination, interference from terrestrial barriers, such as mountains and tall buildings, can be reduced, as the satellite would be more perpendicular to Earth in more areas of the globe.
These are the reasons that prompted the creation of constellations for military and commercial use, such as the Iridium constellation.
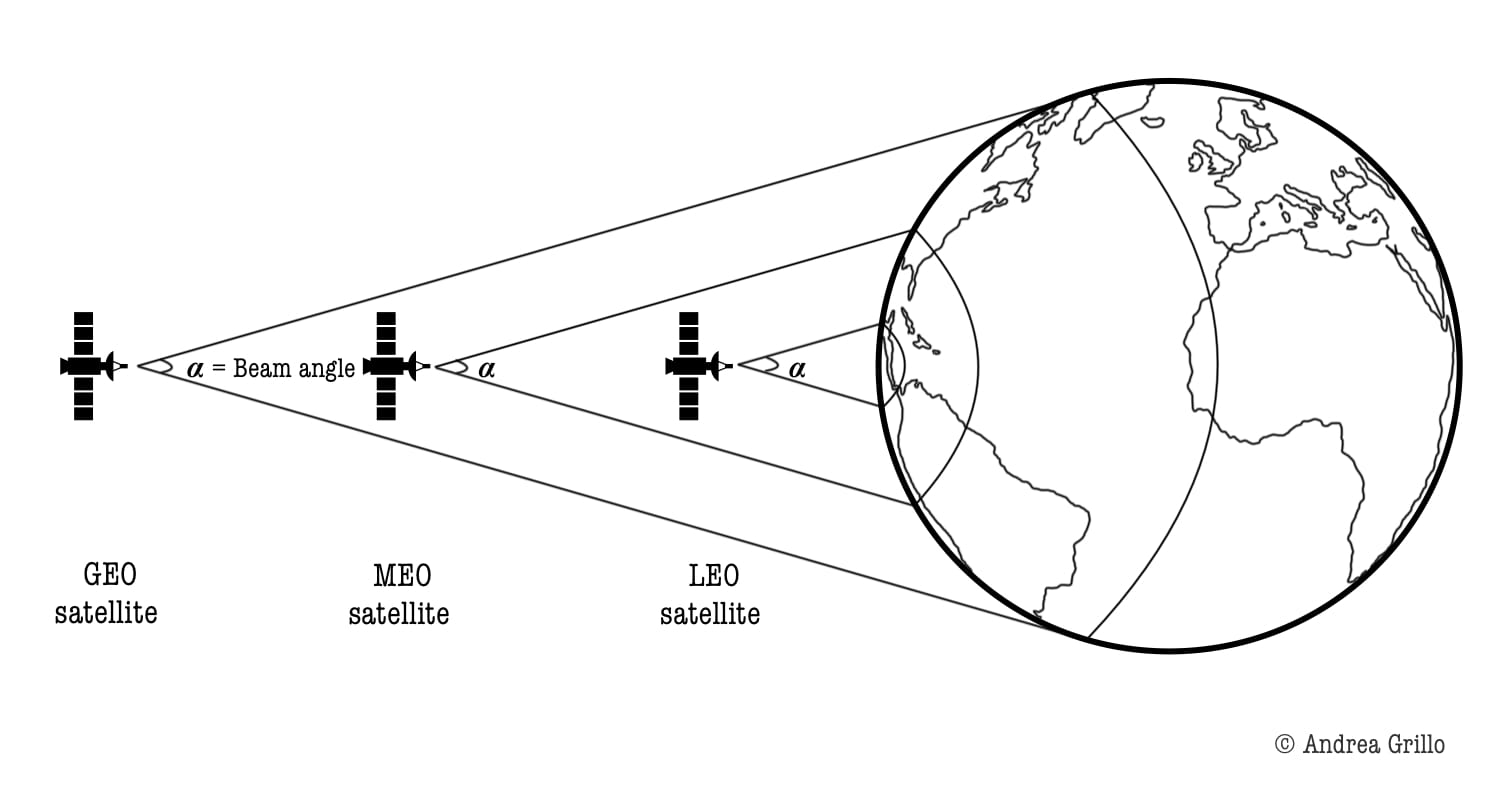
3. The Iridium Constellation
Back in 1998, when its first satellite was launched, the Iridium Constellation was poised to become "the largest satellite constellation in the history of humankind" (Pizzicaroli, 1998, p.113).
Today the Iridium system consists of multiple interconnected satellites strategically positioned around the globe to achieve full Earth coverage, thus enabling communication "anywhere, anytime, and anyplace" (Maine et al., 1995).
Named after the element Iridium which has the atomic number 77 in the periodic table, the network was intended to consist of 77 satellites; however, thanks to optimised orbit trajectories and technological advancements, only 66 active satellites are needed to ensure continuous worldwide coverage (Wright et al., 2005, p.41) (Figure 3).
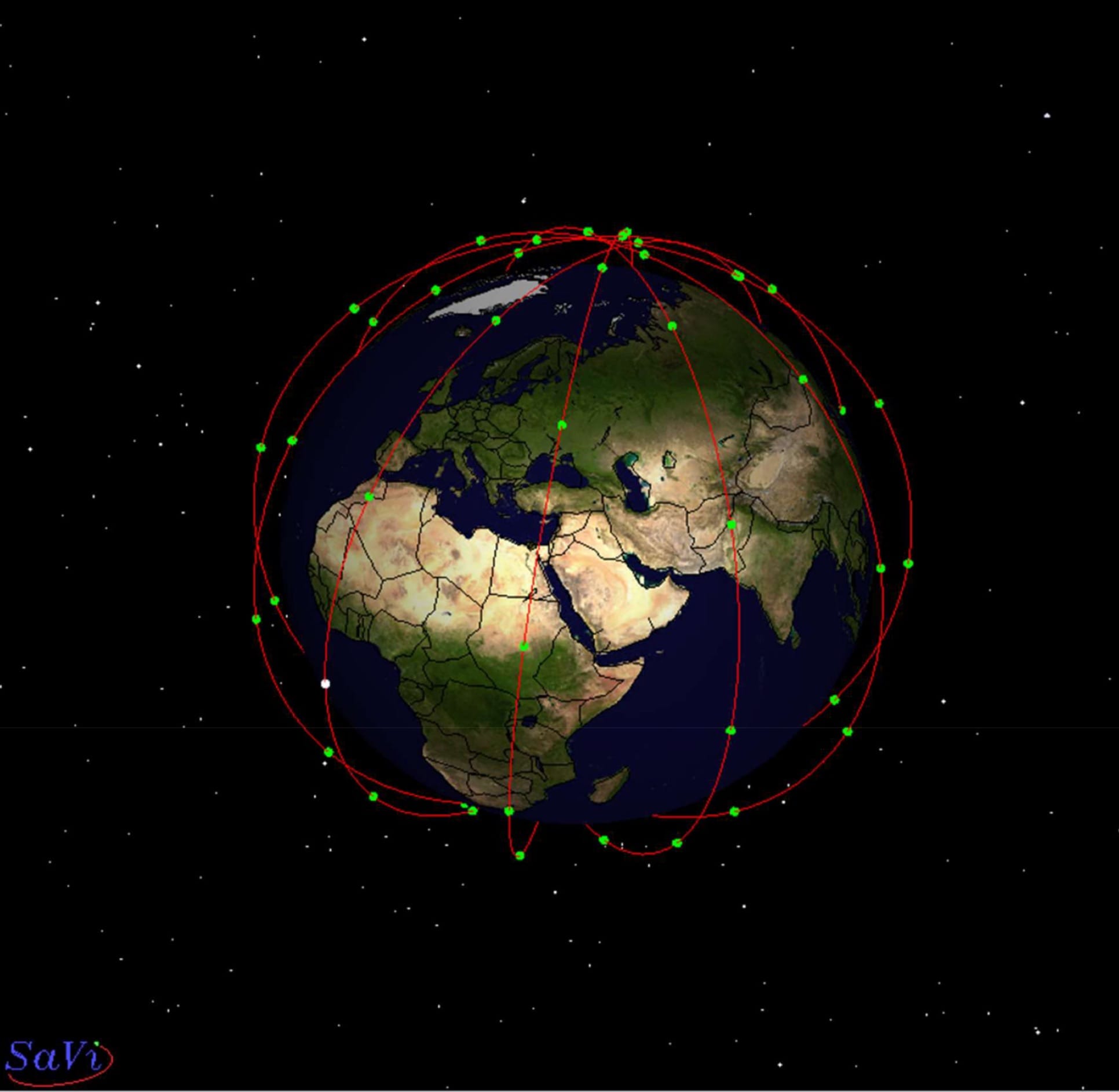
These 66 satellites are positioned at an altitude of about 780 km, orbiting the Earth about every 100 minutes (period = 100 mins), which means that the "average satellite in-sight time to an unmoved terrestrial terminal is around 9 minutes" (Yang, 2020, pp.36-37). Backup satellites in the event of malfunctions are positioned at an altitude of 666 km (Yang, 2020, p.36).
To date, in 2024, Iridium stands as the sole satellite communication network capable of providing true pole-to-pole global coverage for voice, SMS, data, and even broadband services (Iridium Network, n.d. and Yang, 2020, pp.37-38).
With its extensive coverage and robust capabilities, the constellation plays a crucial role in various sectors such as maritime, aviation, government, and emergency services, ensuring reliable connectivity and communication worldwide.
📌 Key Takeaways
- Low Earth Orbit: LEO is defined by altitudes between 100 to 2,000 kilometres. Altitudes above 1,000 kilometres are not widely used due to interference from space weather. Altitudes below 450 kilometres, named Very Low Earth Orbit (VLEO), are increasingly employed owing to advancements in launch systems.
100km<VLEO<450km<LEO<~1000km<space weather
- LEO Operations: LEO primarily serves observation and reconnaissance missions, offering high-resolution imaging and suitability for manned flights. Despite requiring large constellations for continuous coverage, LEO presents advantages for communications such as minimal latency and reduced terrestrial interference.
- The Iridium Constellation: Iridium currently consists of 66 satellites orbiting at approximately 780 kilometres above Earth. This configuration ensures comprehensive global coverage, making Iridium the sole provider of true pole-to-pole global coverage for communications.
References 📃
- Crisp, N. H., Roberts, P. C. E., Romano, F., Smith, K. L., Oiko, V. T. A., Sulliotti-Linner, V., Hanessian, V., Herdrich, G. H., García-Almiñana, D., Kataria, D., & Seminari, S. (2021). System modelling of very low Earth orbit satellites for Earth observation. Acta Astronautica, 187, 475–491. https://doi.org/10.1016/j.actaastro.2021.07.004
- Defense Intelligence Agency (2019). Challenges to Security in Space.
- Dolman, E. C. (2005). Astropolitik: Classical geopolitics in the space age. Routledge.
- Iridium Network. (n.d.). Iridium Satellite Communications. Retrieved 11 April 2024, from https://www.iridium.com/network/
- Maine, K., Devieux, C., & Swan, P. (1995). Overview of IRIDIUM satellite network. Proceedings of WESCON’95, 483-490, IEEE.
- Pizzicaroli, J. C. (1998). Launching and Building the IRIDIUM® Constellation. In J. C. Ha (Ed.), Mission Design & Implementation of Satellite Constellations (Vol. 1, pp. 113–121). Springer Netherlands. https://doi.org/10.1007/978-94-011-5088-0_10
- Wright, D., Grego, L., & Gronlund, L. (2005). The physics of space security. A Reference Manual.
- Yang, X. (2020). Low earth orbit (LEO) mega constellations-satellite and terrestrial integrated communication networks. University of Surrey. https://doi.org/10.15126/thesis.00850382
Keep Learning
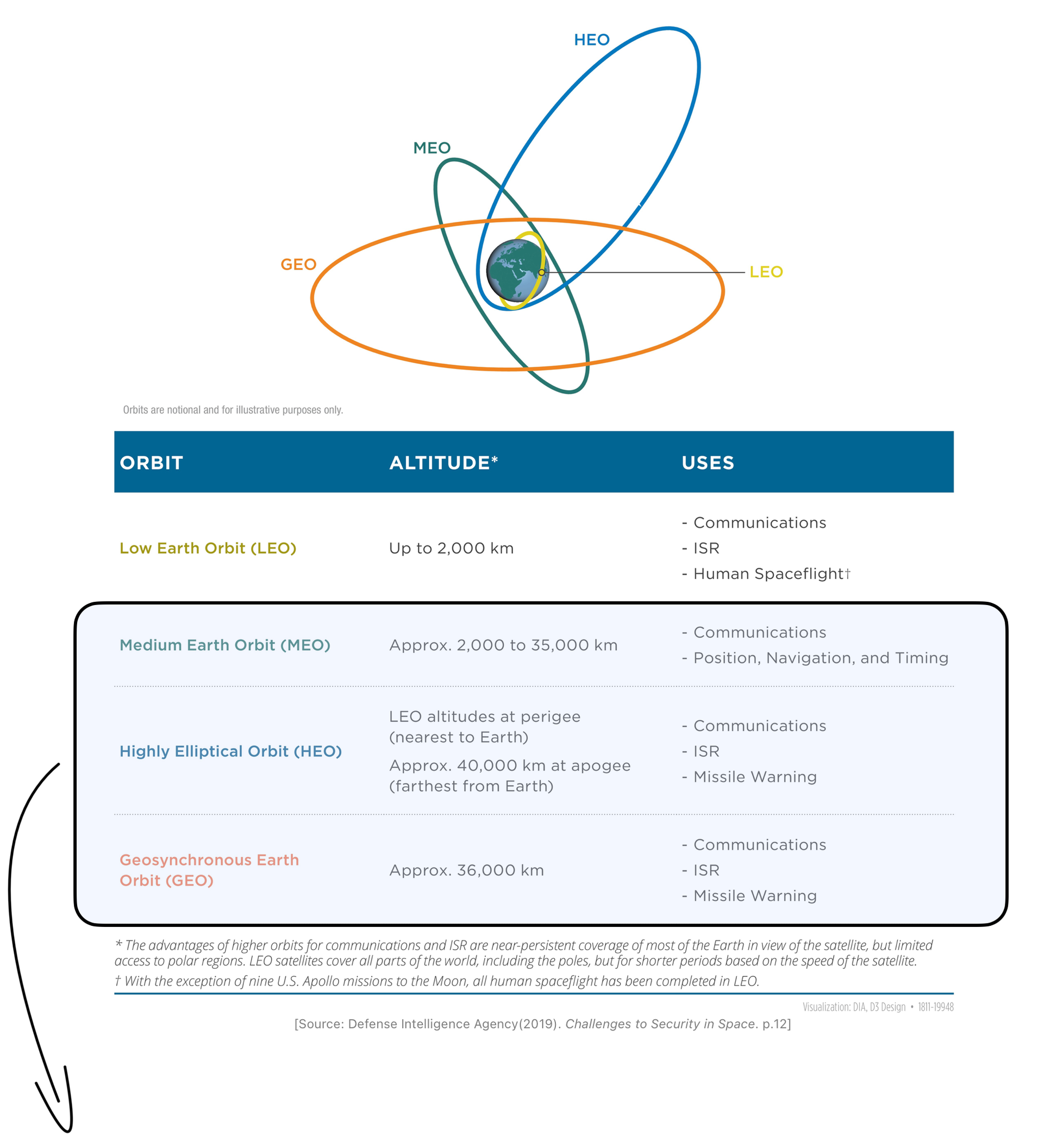